Introduction of the award-wining research
Introduction of the award-wining research
Over the past decade, this group of exceptional scientists conducted frontier laboratory astrophysics that encompasses plasma physics theory, large scale numerical simulations, and experiments on the premier large-scale laser facilities around the world, including the Gekko laser in Japan, Shenguang laser in China, LULI in France, Vulcan in the UK, Large Plasma Device (LAPD) at the University of California, Los Angeles, the Omega and EP lasers at the Univ. of Rochester, New York, and the National Ignition Facility (NIF) at LLNL, in Livermore, CA as shown bin Fig. 1.
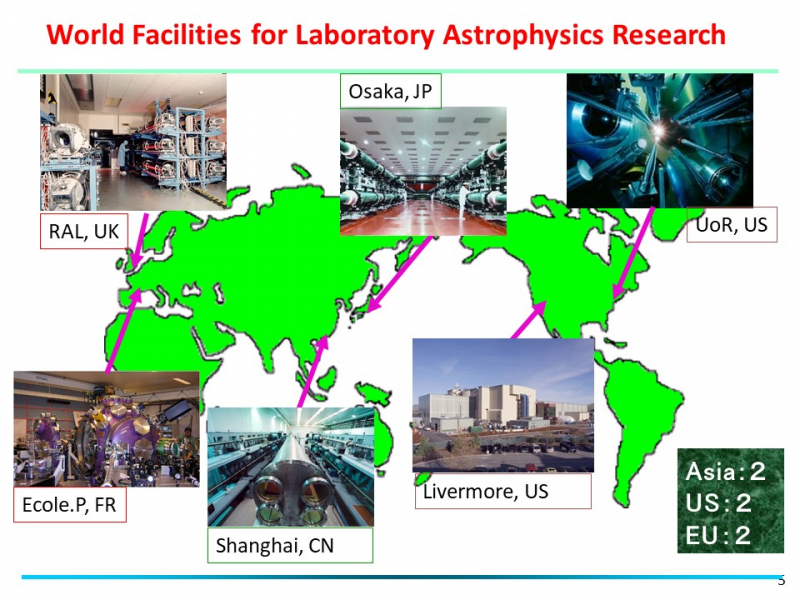
Collisionless shocks are ubiquitous in astrophysical plasmas and have been the topic of intense scientific interest for more than half a century. They are believed to generate and amplify magnetic fields in the universe and accelerate cosmic rays in a variety of objects, including colliding galaxies, proto-stellar jets, supernova remnants, and gamma ray burst, and in high energy density (HED) laboratory experiments. Observations indicate that magnetic fields, either from the ambient medium or self-generated by the shock, are present and important in these systems, hence most astrophysical shocks are electromagnetic in nature, as opposed to electrostatic.
Despite decades of research, it is still not well understood how the structure and evolution of electromagnetic shocks depend on the plasma conditions. The study of these shocks is challenging, due to the need to study the interaction of high-Mach number plasmas that remain collisionless over large system sizes of hundreds of ion skin depths for weakly magnetized plasmas or several ion Larmor radii for magnetized plasmas.
The award-wining international team has studied in depth and at length the microscopic processes that control the structure and evolution of electromagnetic collisionless shocks, including the generation of magnetic fields and turbulence. They created high Mach number plasma flows using high power lasers; then let the plasma interact with either a counter-streaming plasma flow or magnetized plasma. They have extensively documented their work in a series of papers: see list at the bottom. Their key findings can be summarized as follows.
Summary of reported results
[1]. Laboratory observation of the ion-Weibel instability, predicted 50 years ago, which is the fundamental micro-physical mechanism that leads to Weibel-mediated astrophysical collisionless shocks. This demonstrates how Weibel mediated collisionless shocks form and generate magnetic fields, which in astrophysical settings, adds to the magnetic fields of the universe. This work showed definitively both the formation of Weibel filaments in high velocity, interpenetrating plasma flows, and the generation of magnetic fields without any pre-imposed field, at field strengths of a significant fraction of the kinetic energy density. [Fox PRL 2013, Huntington Nature Phys. 2015]
[2]. Laboratory generation of laser-produced magnetized shocks; observation of shock structure, including characteristic shock widths. [Niemann GRL 2014, Schaeffer PRL 2017]
[3]. Experimental, computation, and theoretical demonstration that the Weibel mechanism can work in initially unmagnetized plasmas and lead to a collisionless electromagnetic shocks. [Takabe PPCF 2008, Kato ApJ 2008]
[4]. Observation of ion heating in counter-streaming collisionless plasmas, by the Weibel shock mechanism. [Ross PRL 2013; Ross, PRL 2017]
[5.] Explication of particle acceleration by shocks in both magnetized and Weibel-mediated regimes in astrophysical shocks [Spitkovsky ApJ 2008], and predictions of similar shock structure in laboratory Weibel mediated collisionless shocks [Fiuza PRL 2012, Caprioli ApJ 2014], and first observation of particle injection in laboratory experiments [Rigby Nature Phys. 2018]. In conclusion, it is hard to imagine any team more successful at forging new high-impact, high-visibility science that will set the laser-plasma science standard for future generations. The rigorous connection of this laboratory work to cosmic phenomena such as exploding stars, supernova remnant evolution spanning centuries, bow shocks from proto-stellar jets emitted in star formation, colliding galaxies, and gamma-ray bursts, makes this research stand out as an example of excellence and vision, with broad impact to plasma physics and astrophysics. Such a new field “laboratory astrophysics” shown bin Fig. 2 has grown to a new way to find and predict Astrophysical phenomena in laboratory. And, we believe that it is a new method to challenge advanced plasma physics.
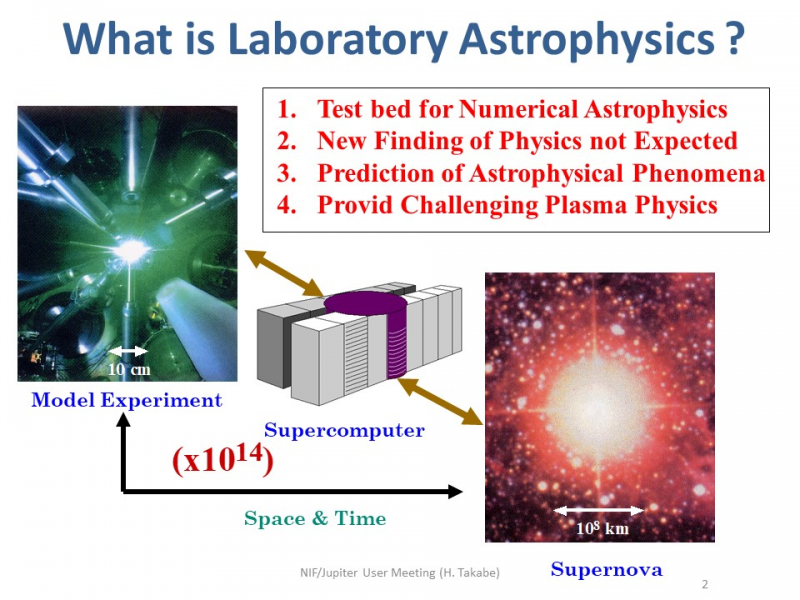
List of the most important publications:
- H. Takabe et al., “High-Mach number collisionless shock and photo-ionized non-LTE plasma for laboratory astrophysics with intense lasers,” Plasma Phys. Control. Fusion 50, 124057 (2008).
- Tsunehiko N. Kato and Hideaki Takabe, “Nonrelativistic collisionless shocks in unmagnetized electron-ion plasmas,” Ap. J. 681, L93 (2008).
- Anatoly Spitkovsky, “On the structure of relativistic collisionless shocks in electron-ion plasmas”, Ap. J. 673, L39 (2008).
- H.-S. Park et al., “Studying astrophysical collisionless shocks with counter-streaming plasmas from high power lasers”, High Energy Density Physics 8, 38 (2012).
- D. D. Ryutov et al., “Basic scalings for collisionless shock experiments”, Plasma Phys. Control. Fusion 54, 105021 (2012).
- J. S. Ross et al., “Characterizing counter-streaming interpenetrating plasmas relevant to astrophysical collisionless shocks”, Phys. Plasmas 19, 056501 (2012).
- F. Fiuza et al., “Weibel-Instability-Mediated Collisionless Shocks in the Laboratory with Ultraintense Lasers”, Phys. Rev. Lett. 108, 235004 (2012).
- R.P. Drake and G. Gregori, “Design considerations for unmagnetized collisionless-shock measurements in homologous flows”, Ap. J. 749, 171 (2012).
- N.L. Kugland, D.D. Ryutov, R.P. Drake, G. Gregori et al., “Self-organized electromagnetic field structures in laser-produced counter-streaming plasmas,” Nature Physics 8, 809 (2012).
- J. S. Ross et al., “Collisionless coupling of ion and electron temperatures in counter-streaming plasma flows”, Phys. Rev. Lett., 110, 145005, (2013).
- W. Fox, G. Fiksel, A. Bhattacharjee, et al., “Filamentation instability of counterstreaming laser- driven plasmas,” Phys. Rev. Lett. 111, 225002 (2013).
- N. L. Kugland, J. S. Ross, R. P. Drake, G. Gregori
- et al., “Visualizing electromagnetic fields in laser-produced counter-streaming plasma experiments for collisionless shock laboratory astrophysics,” Phys. Plasmas, 20, 056313 (2013).
- B. Reville, A. R. Bell, G. Gregori, “Diffusive shock acceleration at laser-driven shocks: studying cosmic- ray accelerators in the laboratory”, New J. Phys. 15 015015 (2013).
- C. Niemann, W. Gekelman, C. G. Constantin, E. T. Everson, D. B. Schaeffer, A. S. Bondarenko, S. E. Clark, D. Winske, S. Vincena, B. Van Compernolle, and P. Pribyl, “Observation of collisionless shocks in a large current-free laboratory”, Geophysical Research Letters 22, 7413 (2014).
- D. D. Ryutov et al., “Collisional effects in the ion Weibel instability for two counter- propagating plasma streams,” Phys. Plasmas, 21, 032701 (2014).
- D. Caprioli and A. Spitkovsky, “Simulations of ion acceleration at non-relativistic shocks. III. Particle diffusion,” Ap. J. 794, 47 (2014).
- C.M. Huntington, F. Fiuza, J.S. Ross et al., “Observation of magnetic field generation via the Weibel instability in interpenetrating plasma flows,” Nature Phys. 11, 173 (2015).
- H. –S. Park et al., “Collisionless shock experiments with lasers and observation of Weibel instabilities”, Phys. Plasmas, 22, 056311 (2015).
- J. S. Ross et al., “Transition from Collisional to Collisionless Regimes in Interpenetrating Plasma Flows on the National Ignition Facility,” Phys. Rev. Lett. 118, 185003 (2017).
- D.B. Schaeffer, W. Fox et al., “Generation and evolution of high-Mach-number laser-driven magnetized collisionless shocks in the laboratory,” Phys. Rev. Lett. 119, 025001 (2017).
- A. S. Bondarenko et al., “Collisionless momentum transfer in space and astrophysical explosions,” Nat. Phys., 13, 573 (2017).
- A. Rigby et al., “Electron acceleration by wave turbulence in a magnetized plasma”, Nat. Phys., March 12 (2018).